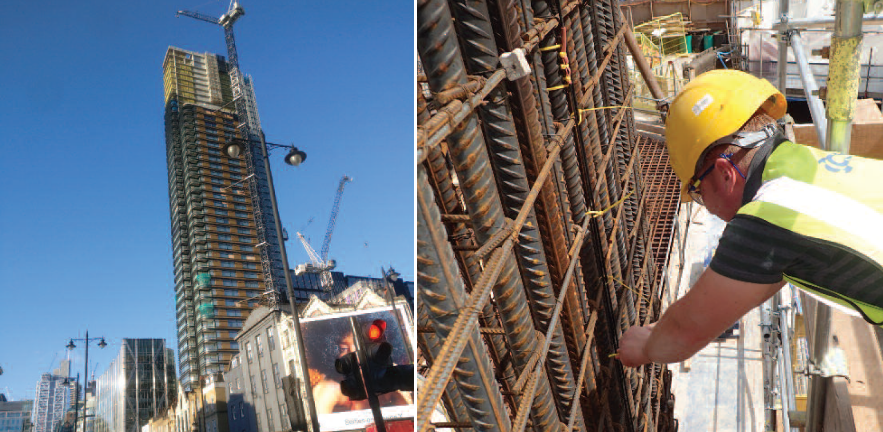
Recent years have seen an increase in proposals, approval and construction of high-rise buildings in the UK, a trend which is set to continue driven by the demand for housing and increased urbanisation. An important factor in the design and construction of high-rise buildings is the axial shortening of vertical load-bearing elements, which is different for each column or wall. Contractors need to allow for the expected final shortening, particularly when setting slab levels and installing finishes and partitions in lower floors, while the building has not yet been completed. However, design predictions of the time-dependent shortening of concrete elements are notoriously inaccurate, as they try to account for the interaction between several parameters. Additionally, these predictions account for the permanent shortening of the members due to the superimposed load and material creep and shrinkage, but do not take into account transient thermal effects.
Conventional monitoring using surveying techniques is limited to measuring shortening at intervals, and at just a few levels. This cannot provide a measure of the local member shortening at un-instrumented levels, or of the global shortening of the whole building. CSIC has developed a novel application of distributed fibre optic sensors (DFOS) to continuously measure the progressive axial shortening of reinforced concrete columns and walls during the construction of high-rise buildings. This monitoring system was successfully trialed for the first time at Principal Tower, a 163 metre-tall, 50-storey residential building in central London, designed by celebrated architectural firm Foster + Partners (Figure 1, pictured above). This trial was carried out in collaboration with the overall project contractor, Multiplex, the frame contractor, Careys, and the structural designers, WSP.
The tower was constructed using an automated jumpform that incorporated the whole building footprint, including the columns. CSIC’s fibre optic (FO) strain and temperature sensing cables were continuously embedded inside two columns and two locations in the core walls of Principal Tower, as construction progressed. The FO cables were prepared in advance with sufficient length to extend all the way up to the top of the building, and wound on cable reels that were housed in the jumpform rig. This enabled the rig operatives to un-reel the cables as the construction progressed upwards. The FO cables were tied to the reinforcement of the instrumented elements during steel fixing, and subsequently embedded in the concrete (Figure 2, pictured above). CSIC trained the contractor’s operatives to install the FO cables, and CSIC researchers analysed the data and provided the required information to the contractor and design engineers.
Monitoring from the DFOS system started in September 2016, just before the ground floor of the tower was concreted. Since then, measurements have been taken at least twice every hour. Each measurement consists of strain and temperature readings from around 3,000 points along the height of the four instrumented locations. These data were used to calculate the axial shortening relative to the ground level, along the whole height of the completed elements, at any time during the construction. This enabled the engineers and contractors to verify their predictions and adjust their assumptions if necessary, thus benefiting the construction sequence.
Results
The axial shortening of the columns derived from the monitoring system during the first 10 months of construction are shown in Figure 3 (download the full case study to see the figure). Except for some periods where the monitoring was stopped to enable repairs or alterations to be made to the monitoring system, the data provide a continuous profile of the building’s shortening over time. This has enabled the project team to make a number of observations.
Differential shortening
The four instrumented elements have deformed at different rates. Column 1, which has approximately half the cross-sectional area of column 2, has shortened around 15–25 per cent more than column 2, despite supporting approximately 12 per cent less dead load. Similarly, both columns have shortened significantly more than the core walls, as the walls have a larger cross-sectional area and are stiffer. This was expected, since an element’s shortening is related to its cross-sectional area and surface area. This observation nevertheless highlights the importance of accounting for the differential shortening across the footprint of a tall building.
Thermal effects
The data show a strong thermal effect that gives rise to occasional fluctuations in the axial movement of each instrumented element, due to expansion and contraction of the building. One example is the decrease in shortening that happened due to expansion of the building during a warm period in mid-June 2017. By estimating the expansion and contraction of the concrete elements, it is possible to derive the axial shortening due to mechanical effects alone. This showed that thermal effects accounted for as much as half of the total deformation during temperature extremes. Such thermal effects can have unexpected consequences when using shortening estimates to pre-set column heights, particularly when concreting during hot or cold periods, since these estimates do not normally account for transient thermal effects.
DFOS monitoring system offers unprecedented detail
The data acquired to date provide the shortening time histories of the instrumented elements with unprecedented detail and at an unprecedented temporal density. This information has been used to demonstrate how an element’s shortening is affected by its profile and stiffness, with smaller and less stiff elements shortening more. The continuous data also show that transient thermal effects can play a significant role in axial shortening, at times accounting for as much as 50 per cent of the total deformation. This is particularly significant as shortening predictions prior to construction do not take into account such thermal effects. CSIC’s FO monitoring system also makes it possible to observe the effects of occasional and unexpected events – such as an incident of abrupt loading – which could not be observed with periodic or occasional measurements.
Future prospects
This FO monitoring system provides continuous data throughout construction, enabling engineers and contractors to verify predictions and adjust assumptions if necessary. No other monitoring system is able to provide this information with such spatial and temporal density. Design engineers and contractors working on the construction of other tall buildings in the future would benefit from using this monitoring system, which provides valuable insight into a problematic phenomenon that is otherwise very difficult to quantify precisely. This knowledge can lead to more efficient and cost-effective construction of high-rise buildings.
The DFOS-based axial shortening monitoring system developed by CSIC can also be applied to other long and/or slender structures, where thermal effects, load and material properties can lead to significant axial movement, such as in bridges and large area concrete slabs.
Project contact Dr Nicholas de Battista, Research Associate